Nature: Design principles of nanoparticles to overcome the biological barrier of drug delivery
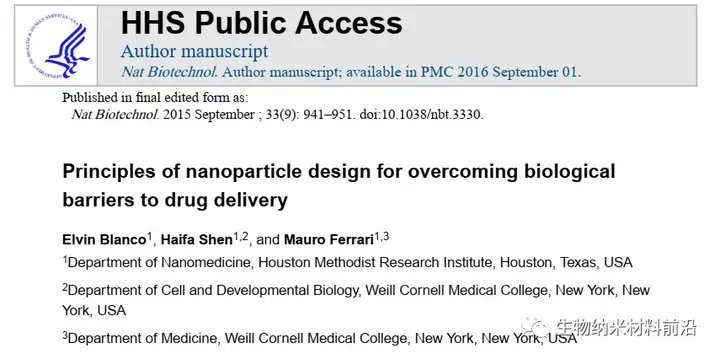
1. Article overview
In the treatment of various diseases, whether patients can be successfully cured depends on the ability of doctors to deliver drugs to specific sites. Cancer is a typical example. After a century of development, today’s treatment methods still cannot concentrate drugs. At the site of action of the drug, the drug molecule simply spreads throughout the body, which can easily cause adverse side effects in the human body and waste a lot of drugs. In the long-term research on drug delivery, scholars have found that nanoparticles have become a suitable carrier to overcome the limitations of conventional drug formulations and their related pharmacokinetics. For example, liposomes have been proven to have advantages in dissolving therapeutic drugs and can control the long-term drugs. Sustained release greatly prolongs the circulating life of the drug. However, the biological barrier in the drug delivery process prevents the accumulation of nano-drug carriers in the diseased part, thereby limiting the effective response during drug treatment. Although a large amount of research work aims to incorporate multiple functions into the entire nanoparticle design, many of these strategies have failed to adequately address these obstacles, such as non-specific distribution and insufficient accumulation of therapeutic drugs, which are still huge challenges for drug developers. Unless the design of nanocarriers can solve most (if not all) biological barriers encountered by particles during intravenous administration, the delivery of therapeutic drugs to specific sites will still be a distant reality. Therefore, we can design nanoparticles for the biological barriers that appear in drug delivery, and rationally combine innovative design features. This will create a new generation of nano-therapeutic drugs and realize the successful transformation of nanoparticle-based drug delivery.
Two, graphic guide
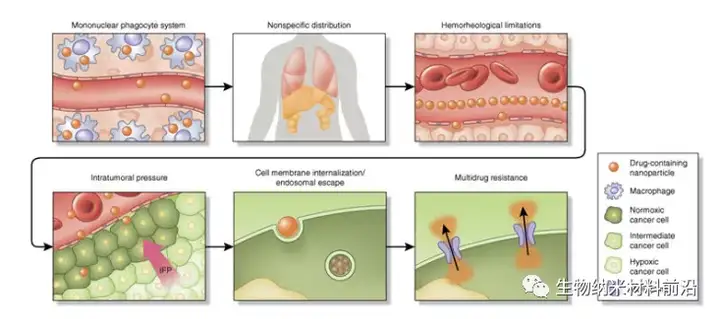
Figure 1. Continuous biological barrier framework for nanoparticle drug delivery. When administered intravenously, drug-containing nanoparticles encounter many site-specific delivery barriers that prevent effective. The nanoparticles undergo opsonization and are then taken up by the resident macrophages of MPS. This will cause the nanoparticles to accumulate in organs (such as the spleen and liver), leading to non-specific distribution of nano-therapeutic drugs to healthy organs. Under normal flow conditions of blood vessels, the size and geometry of nanoparticles have a great influence on the edge dynamics of the blood vessel wall. Small-sized spherical particles migrate in the cell-free layer, quite far from the endothelial surface, limiting active targeting strategies and effective accumulation through passive targeting mechanisms (such as EPR). Another important obstacle to the accumulation of nanoparticles in tumors is high intratumoral pressure, which is caused by disrupted vasculature, aggressive cell growth, fibrosis, dense extracellular matrix and damaged lymphatic vessels. Cell internalization and endosomal escape are another powerful obstacle. The size and surface modification of nanoparticles affect their internalization pathways (such as clathrin and caveolin) and intracellular flow. Under low pH environment and enzyme action, the endosomal compartmentalization of internalized nanoparticles proved to be harmful to cells, especially genetic material. The last obstacle is that after the nanoparticles enter the cell, the drug efflux pump that confers treatment resistance expels the chemotherapeutic drug from the cell. IFP and interstitial fluid pressure play a key role in it.
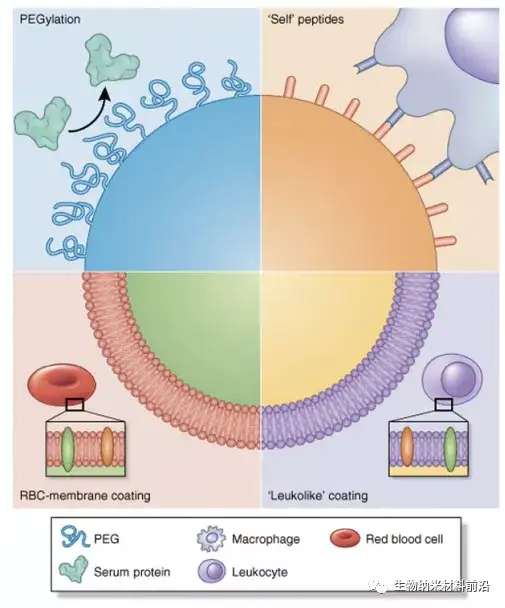
Figure 2. Nanoparticle biomimetic strategies used to avoid MPS and prolong circulation. The conditioning effect and fit of MPS have been shown to be detrimental to the long cycle time of nanoparticles. Therefore, we have used several strategies to "disguise" nanoparticles and prevent protein adsorption. Pegylation represents a classic strategy in which PEG is grafted onto the surface to provide a hydration layer that prevents the formation of protein corona. In another strategy, the CD47 peptide is attached to the surface of the nanoparticle, and then the macrophage recognizes the nanoparticle as "self" so that the nanoparticle avoids phagocytosis. Finally, coating the nanoparticles with cell membranes extracted from autologous white blood cells and red blood cells (RBC) provides a biomimetic surface that significantly prolongs the circulation of nanoparticles in the body.
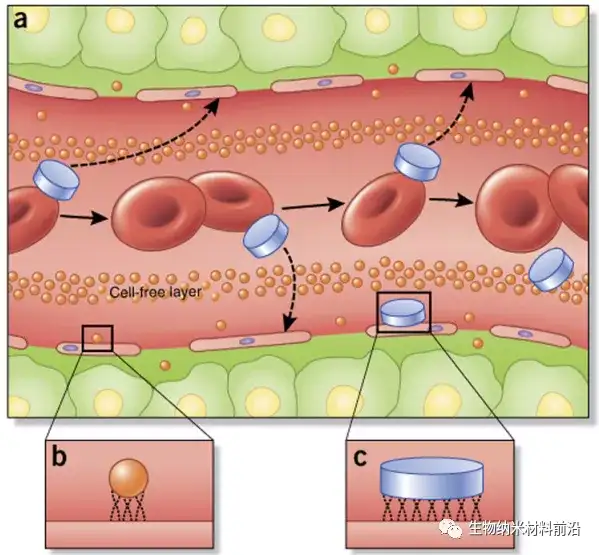
Figure 3. The flow, edge, and adhesion characteristics of nanoparticles in blood vessels depend on particle size and geometry. (a) Unlike spherical nanoparticles, non-spherical particles, such as those with a disc-like geometry, are more likely to produce tumbling and oscillation effects in the vasculature, which greatly increases the tendency of nanoparticles to contact the cell wall and pass through the vasculature. The windows in the system may leak. (b,c) Once in contact with endothelial cells, the small size and surface area of conventional spherical nanoparticles (b) Compared with larger disc-shaped nanoparticles (c; and other non-spherical geometries), it reduces binding and contact points This will affect tumor accumulation and active targeting strategies.
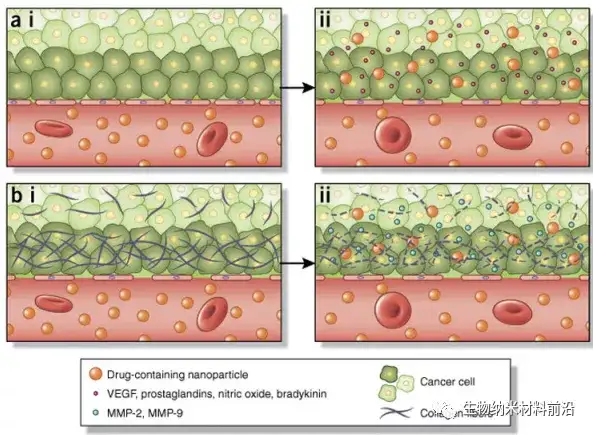
Figure 4. The determinants of nanoparticle-induced enhancement of tumor tissue permeability. EPR effect 3 is a transport phenomenon, and its main feature is the existence of windows in the tumor vasculature, which enables the passive accumulation of nanoparticles in the tumor. (a,i) Normal vasculature usually has tight interendothelial connections, which can prevent extravasation of particles into tissues. The imbalance of several factors will affect the size of the window in the tumor vascular system. (a,ii) For example, VEGF and nitric oxide have been shown to increase the size of the endothelial cell gap. (b,i) Tumors usually have a dense extracellular matrix that prevents nanoparticles from fully infiltrating the tumor. (b,ii) MMP-2 and MMP-9 degrade the dense collagen matrix that constitutes the basement membrane. In turn, these can be used pharmacologically (for example, by administering anti-fibrotic agents) to enhance the EPR effect, thereby increasing the accumulation of nanoparticles in tumors.
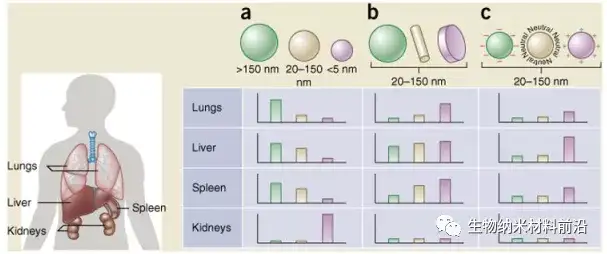
Figure 5. The size, shape, and surface charge of nanoparticles determine their biodistribution among different organs (including lung, liver, spleen, and kidney). (a) Spherical particles, including gold nanoparticles, liposomes, and polymer micelles/nanoparticles, may have different sizes and show different flow directions in the body. Large rigid particles with a diameter> 2,000 nm are easy to accumulate in the capillaries of the spleen and liver and lungs; nanoparticles with a diameter in the range of 100-200 nm leak out through the vascular window of the tumor (EPR effect) and escape the liver and spleen As the size increases to more than 150 nm, more and more nanoparticles are encapsulated in the liver and spleen; small size nanoparticles (<5 nm) are filtered out by the kidney. (b) New "top-down" and "bottom-up" manufacturing technologies make it possible to explore the different geometric shapes of nanoparticles, including cylindrical and disc-shaped shapes, which have shown their impact on pharmacokinetics and biodistribution Significant impact. Different nanoparticle shapes exhibit unique flow characteristics, which significantly change the cycle life, cell membrane interaction and macrophage uptake, which in turn affects the biodistribution between different organs. (c) The charge of nanoparticles derived from different surface chemicals affects conditioning, circulation time, and interaction with resident macrophages of organs containing MPS. Positively charged particles are more likely to be absorbed in the lungs, liver, and spleen Isolation of macrophages. Neutral and slightly negatively charged nanoparticles have a longer cycle life and less accumulation in the aforementioned organs of MPS. In b and c, the hypothetical nanoparticle size range is 20-150 nm. It is worth noting that the biological distribution in the body will undoubtedly change according to the interaction of the above-mentioned parameters.
3. Full text summary
At present, the field of drug delivery of nanoparticles is going beyond the traditional scope (for example, traditional geometry, size or chemical substances), which facilitates the design of nanoparticles that are specifically responsible for overcoming continuous biological obstacles. People are increasingly aware that although nanoparticles are indeed complex for the biological barriers in the treatment of tumors, they are by no means insurmountable. As emphasized here, the implementation of innovative designs, such as the use of non-traditional geometric shapes to improve vascular dynamics or the use of biomimetic membrane functionalization to avoid uptake by phagocytes, has shown clear advantages over existing conventional nanoparticles. Although the field is thinking about more reasonable ways to overcome biological obstacles, these should revolve around the additional complexity associated with these systems, which will directly affect the ease of scale, large-scale production and related costs. In addition, depending on the design and implementation (for example, the addition of a biomimetic surface derived from autologous cells), regulatory approvals related to quality control, repeatability, and toxicity may be additional obstacles. A better understanding of the control of these biological barriers and their transformation in various disease states, coupled with innovations in materials science, will continue to promote the development of nanoparticles that can solve these barriers to achieve effective, site-specific Delivery. This will not only lead to the successful transformation of new therapies, but also elevate nanoparticle-based drug delivery from a promising field to a feasible and common strategy for the treatment of multiple diseases.
At present, the field of drug delivery of nanoparticles is going beyond the traditional scope (for example, traditional geometry, size or chemical substances), which facilitates the design of nanoparticles that are specifically responsible for overcoming continuous biological obstacles. People are increasingly aware that although nanoparticles are indeed complex for the biological barriers in the treatment of tumors, they are by no means insurmountable. As emphasized here, the implementation of innovative designs, such as the use of non-traditional geometric shapes to improve vascular dynamics or the use of biomimetic membrane functionalization to avoid uptake by phagocytes, has shown clear advantages over existing conventional nanoparticles. Although the field is thinking about more reasonable ways to overcome biological obstacles, these should revolve around the additional complexity associated with these systems, which will directly affect the ease of scale, large-scale production and related costs. In addition, depending on the design and implementation (for example, the addition of a biomimetic surface derived from autologous cells), regulatory approvals related to quality control, repeatability, and toxicity may be additional obstacles. A better understanding of the control of these biological barriers and their transformation in various disease states, coupled with innovations in materials science, will continue to promote the development of nanoparticles that can solve these barriers to achieve effective, site-specific Delivery. This will not only lead to the successful transformation of new therapies, but also elevate nanoparticle-based drug delivery from a promising field to a feasible and common strategy for the treatment of multiple diseases.
Article link:
Doi: 10.1038/nbt.3330.
Doi: 10.1038/nbt.3330.
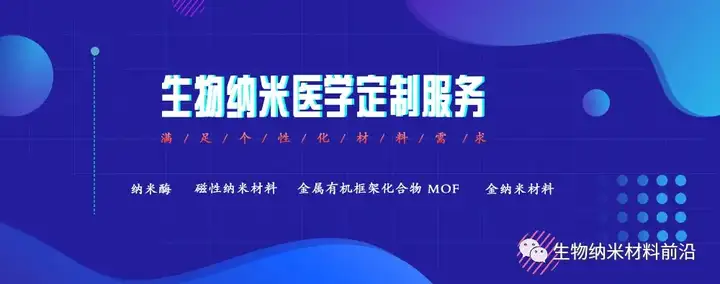
This information is sourced from the Internet for academic exchanges only. If there is any infringement, please contact us to delete it immediately.
18915694570
Previous: Applied Catalysis B: E