3D bioprinting of granular hydrogels containing platelet derivatives
Tissues have a hierarchical structure, consisting of a dynamic extracellular matrix with biological activity and multiple cell populations. The extracellular matrix components are interconnected to establish unique components and topological structures, provide structural and biochemical support for cells, and regulate cell behavior (such as proliferation, growth, migration, and differentiation). In addition, the extracellular matrix can also dynamically and locally regulate the release of soluble and immobilized molecules. Due to its hydration network and unique physical and chemical properties, hydrogels can regulate cell behavior and are often used as mimics of extracellular matrix. Microgel is a new type of ink prepared by a bottom-up engineering strategy. Compared with traditional bulk hydrogels, microgels have significant porosity, increased specific surface area, modularity and excellent controllable mechanical response.
Recently, the team of Professor Jason A. Burdick from the Department of Bioengineering of the University of Pennsylvania in the United States combined photocrosslinkable norbornene-modified hyaluronic acid (NorHA) with human platelet lysate (PL), and combined microfluidic technology to prepare Spherical PL-NorHA microgel with hierarchical fiber network. This kind of microgel has shear thinning and self-healing properties, and can be used to create a stable 3D structure through 3D printing technology. Related research paper: "Injectable hyaluronic acid and platelet lysate-derived granular hydrogels for biomedical applications" was published on Acta Biomaterialia.
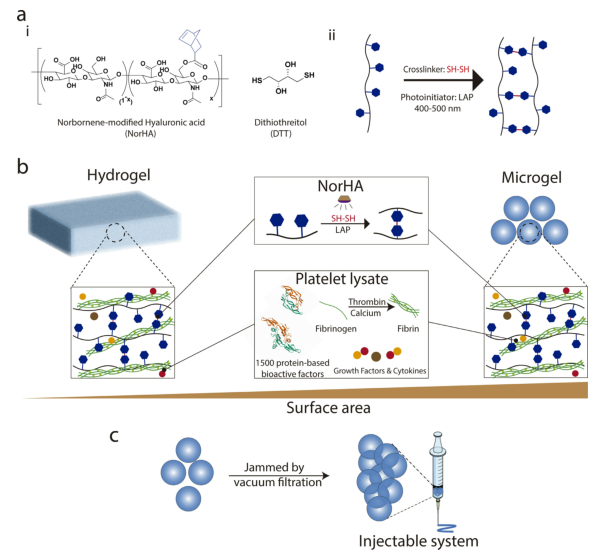
Figure 1 Preparation of PL-NorHA hydrogel and microgel
Gel material: photocrosslinkable norbornene modified hyaluronic acid (NorHA)
Process: Synthesis of PL-NorHA hydrogel: NorHA and PL matrix are incubated at room temperature for 20 minutes, and then cured with visible light for 5 minutes to form PL-NorHA hydrogel.
Preparation of PL-NorHA microgel: Precursor 1 (NorHA or NorHA containing coagulation factors) and precursor 2 (PBS or PL) were introduced into the microfluidic device at flow rates of 1μl/min and 2μl/min, respectively. At the exit (≈28 cm), the gel is activated and then cured by visible light to form polymer droplets.
1. Characterization of PL-NorHA hydrogel In order to analyze the fiber structure of PL-NorHA hydrogel, the researchers added fluorescent fibrinogen to the PL solution and observed it with a confocal microscope. Figures 2A-i,ii show that when the NorHA concentration is 1% and 2%, the hydrogel shows interconnected and uniform fiber structure similar to a typical layered fiber matrix. The introduction of this multi-scale attribute reproduces the complexity of the hierarchical organization of natural ECM from nanoscale to microscale. In order to better understand the gel dynamics and network characteristics of the obtained hydrogels, the researchers analyzed their gel process and viscoelastic properties (Figure 2b-i~iv). Before visible light irradiation (20min), NorHA, NorHA protein (ie without coagulant) and PL-NorHA (ie with coagulant) have lower storage modulus, and their mechanisms are similar (G≈1-2Pa and G≈0.4-1Pa). However, when exposed to visible light, due to the covalent photo-crosslinking reaction of the thiol-ene reaction, the elastic modulus and viscous modulus increase and reach the storage modulus plateau within a few seconds (2b-i-iv) . PL-NorHA hydrogel (G: 593±156Pa-1wt.% and G: 3536±159Pa-2wt.%) contains only NorHA (G: 1655±238Pa-1wt.% and G: 4845± 376Pa-2wt.%) or NorHA hydrogels containing protein (G: 1561±75Pa-1wt.% and G: 5324±722Pa-2wt.%) have lower storage modulus.
2. Release of protein in PL-NorHA hydrogel. The biosynthesis and tissue turnover of HA are regulated by synthetase and hyaluronidase. The researchers used the quantification of uronic acid or total protein in the hydrogel to draw the in vitro degradation curve of the hydrogel (Figure 2C). Studies have found that in the presence of hyaluronidase, the total degradation time and degradation rate of the hydrogel are faster. There is also a correlation between the degradation time and the crosslink density. The degradation rate of high concentration (2wt.%) is slower than that of low concentration (1wt.%). In addition, the degradation rate of PL-NorHA hydrogel is faster than NorHA (Figure 2c-i,iii). This is because PL-NorHA hydrogels, in addition to the covalent photo-crosslinked network (NorHA), also contain a cross-linked PL protein-bound fiber matrix. Therefore, the degradation of PL-NorHA hydrogel is not only due to the release of uronic acid (representing HA degradation), but also related to the degradation of the fibrin matrix. 1wt.% PL-NorHA hydrogel (92±1%) has higher PL-derived protein release than 2wt.% PL-NorHA hydrogel (72±2%). These results indicate that different combinations of HA macromolecule concentration and fibrin matrix can regulate the release of drugs in drug-loaded hydrogels.
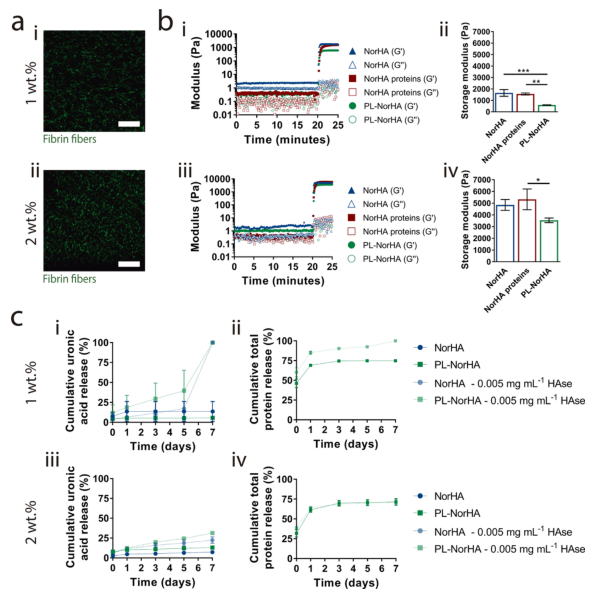
Figure 2 Characterization of the mechanical properties and degradation properties of PL-NorHA hydrogels 3. The in vitro response of MSCs to PL-NorHA hydrogels. Researchers inoculated MSCs cells in NorHA, NorHA-RGD and PL-NorHA under serum-free conditions On the hydrogel, the cell morphology was observed after 4h and 1d in vitro culture. The cells seeded on the NorHA hydrogel usually exhibit a round morphology, with some protrusions at the concentrations of 1wt.% and 2wt.%, showing comparable cell spreading areas and aspect ratios over time (Figure 3a) . The 1wt.% and 2wt.% NorHA-RGD hydrogels can greatly increase the cell spreading area. In PL-NorHA hydrogels (1wt.% and 2wt.%), the cells adhered, spread and presented a spindle-like morphology after only 4 hours of culture (Figure 3a, b). After culturing for 1 day, MSCs showed slender protrusions and formed interconnected networks with neighboring cells. These changes may be caused by the fibrous network formed by PL-NorHA hydrogel. It not only provides a temporary hierarchical extracellular matrix, giving mechanical support and extracellular matrix topology, but also contains many binding domains that mediate interactions with cells, extracellular matrix proteins and growth factors.
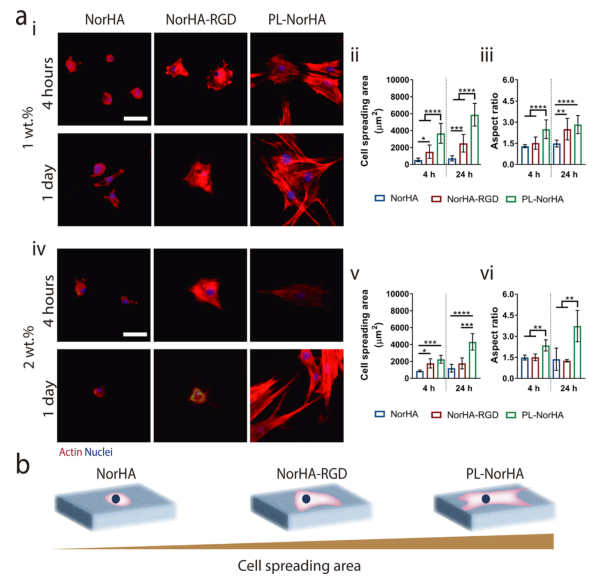
Figure 3 Evaluation of in vitro cell viability of PL-NorHA hydrogel
4. Preparation and characterization of microgels. NorHA (coagulation factor) and PL containing coagulation factors are introduced into the Y-shaped microfluidic chip through two independent channels at different flow ratios, and they are converged into a laminar flow channel. Promote the polymerization of fibrin. Then, this single channel is interrupted by the incompatible continuous phase, producing water-in-oil droplets. When passing through the serpentine path, fibrin further polymerizes and cross-links with visible light at the end of the channel to form a microgel (Figure 4a). Researchers incorporated fluorescein-labeled glucose during the formation of the microgel to analyze its morphology (including size and roundness) (Figure 4b, c). PL-NorHA microgels produced a uniform and layered fibrin network (Figure 4b-ii). The average diameters of the spherical microgels are 120±12μm (1wt.%NorHA), 126±17μm (1wt.%PL-NorHA), 100±8μm (2 wt.%NorHA) and 119±21μm (2 wt.%). PL-NorHA) (Figure 4c-i,ii). Through the release of uronic acid and total protein, the researchers assessed the degradation of the microgel. Studies have found that microgels show a tendency to degrade in the presence of hyaluronidase, and that microgels with low concentrations degrade faster than microgels with high concentrations; PL-NorHA microgels degrade faster than NorHA microgels The gel is faster; the degradation rate of the microgel state is faster than that of the hydrogel state (Figure 4d-i, iii). The total protein release was quantified. It was found that 7 days later, with or without hyaluronidase, the total protein release of PL-NorHA was 41%±7% and 69%±3%, respectively. In summary, it is shown that the stability of the microgel and the release rate of treatment-related proteins can be adjusted by changing the cross-linking density and the concentration of hyaluronidase.
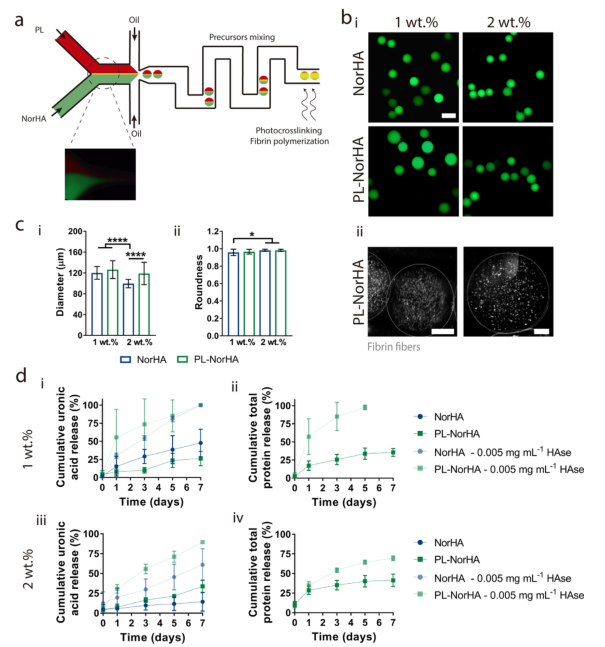
Figure 4 Preparation and characterization of PL-NorHA microgel
5. The rheological properties of the particulate hydrogels In order to study the printability of the microgels, the researchers evaluated the rheological properties and structure of the microgels (Figure 5). The experimental results of oscillating shear rheology show that when the concentration of NorHA polymer in the microgel increases from 1% to 2%, the storage modulus of the particulate hydrogel increases by an order of magnitude (Figure 5a-I, iv). Consistent with the observations in the hydrogel, the presence of PL significantly reduced the storage modulus of the granular hydrogel (from 77±3Pa to 234±2Pa, 2wt.% NorHA and PL-NorHA, respectively). The microgel has shear thinning and self-healing rheological properties, and can be extruded with minimal force without changing the initial properties. The granular hydrogels prepared from different concentrations of microgels (1wt.% and 2wt.%) all showed shear yield with increasing strain (Figure 5a-ii, v). Through a series of high (500%) and low (0.5%) strain scans, the self-healing behavior of all particle hydrogels was observed. After being subjected to high strain, the material showed rapid recovery of solid-like behavior (Figure 5a-iii, vi). In order to better observe the structure of the particulate hydrogel, the researchers incubated the microgel with rhodamine thiol for visualization before making water-in-oil droplets. The results show that 1wt.% NorHA and PL-NorHA microgels show very small porosity, while 2wt.% PL-NorHA microgels increase the porosity, and 2wt.% NorHA microgels have the largest porosity. This may be due to the viscoelasticity of the microgel. The low mechanical extrusion of microgels increases their bulk density and reduces the surface area (Figure 5B).
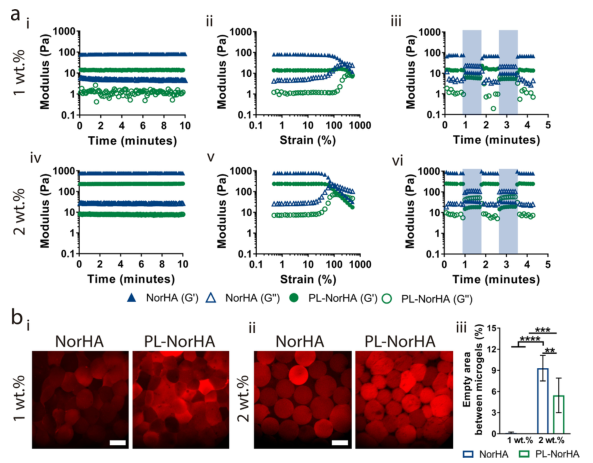
Figure 5 Rheological and morphological characterization of PL-NorHA granular hydrogel
6. 3D printed particle hydrogel and its in vitro cell response In order to evaluate the printability of the microgel ink, the researchers printed the microgel containing FITC-dextran on the surface of the glass slide in filament form, and used fluorescence Microscopic observation (Figure 6a). Due to its granular structure, the microgel ink is easily extruded to form uniform filaments, presenting a densely packed microgel morphology. Furthermore, the researchers used microgel inks for 3D printing to create 3D structures (Figure 6b-i). The lower concentration of microgel ink produces a stable 3D structure (Figure 6b-ii, iii, v), and the higher concentration of NorHA microgel produces a structure that is easily destroyed (Figure 6b-iv). In addition to structural integrity, the printed structure also shows high fidelity. Then the researchers used 1wt.% NorHA and PL-NorHA microgels loaded with MSCs cells for 3D printing. MSCs grown on NorHA microgels showed a round shape (Figure 6c-i), similar to the results obtained from NorHA hydrogels; while the interconnected micropore spaces in PL-NorHA microgels promoted MSCs cells The massive migration and the formation of a 3D cell network (Figure 6c-i, iii). This indicates that PL-derived proteins can promote cell adhesion and survival, and can form a hierarchical fibrin network that can provide cell anchor points.
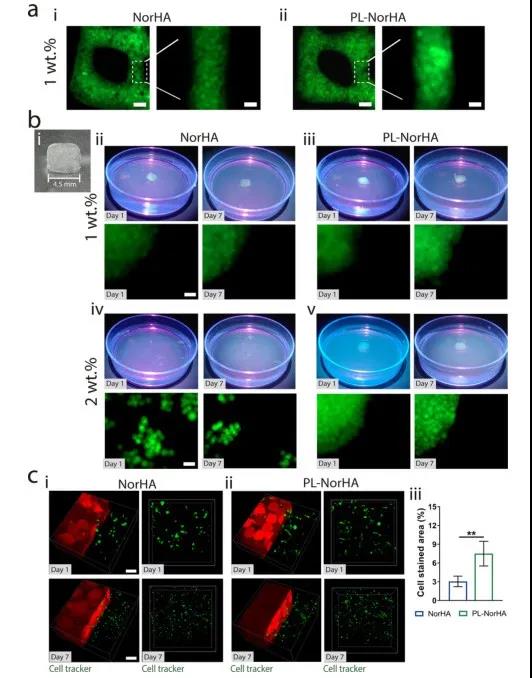
Figure 6 3D printing structure stability and in vitro cell viability evaluation of PL-NorHA microgel.
7. Summary The researchers photo-crosslinked norbornene-modified hyaluronic acid (NorHA) and human platelet lysate (PL) to prepare PL-NorHA hydrogel ink. PL-NorHA hydrogel ink can adjust the release of PL-derived proteins by changing the concentration of HA, and the formed hierarchical fibrin network can promote the adhesion and migration of MSCs cells. In addition, the PL-NorHA microgel ink constructed based on microfluidic technology shows shear thinning and self-healing properties, and can be used for minimally invasive drug delivery and 3D bioprinting.
Recently, the team of Professor Jason A. Burdick from the Department of Bioengineering of the University of Pennsylvania in the United States combined photocrosslinkable norbornene-modified hyaluronic acid (NorHA) with human platelet lysate (PL), and combined microfluidic technology to prepare Spherical PL-NorHA microgel with hierarchical fiber network. This kind of microgel has shear thinning and self-healing properties, and can be used to create a stable 3D structure through 3D printing technology. Related research paper: "Injectable hyaluronic acid and platelet lysate-derived granular hydrogels for biomedical applications" was published on Acta Biomaterialia.
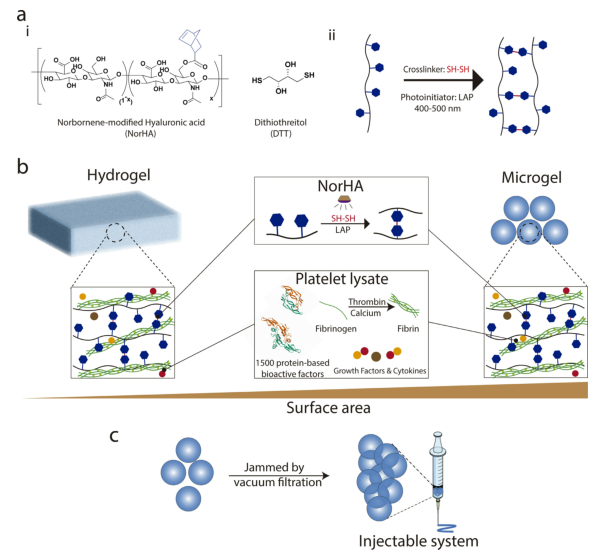
Figure 1 Preparation of PL-NorHA hydrogel and microgel
Gel material: photocrosslinkable norbornene modified hyaluronic acid (NorHA)
Process: Synthesis of PL-NorHA hydrogel: NorHA and PL matrix are incubated at room temperature for 20 minutes, and then cured with visible light for 5 minutes to form PL-NorHA hydrogel.
Preparation of PL-NorHA microgel: Precursor 1 (NorHA or NorHA containing coagulation factors) and precursor 2 (PBS or PL) were introduced into the microfluidic device at flow rates of 1μl/min and 2μl/min, respectively. At the exit (≈28 cm), the gel is activated and then cured by visible light to form polymer droplets.
1. Characterization of PL-NorHA hydrogel In order to analyze the fiber structure of PL-NorHA hydrogel, the researchers added fluorescent fibrinogen to the PL solution and observed it with a confocal microscope. Figures 2A-i,ii show that when the NorHA concentration is 1% and 2%, the hydrogel shows interconnected and uniform fiber structure similar to a typical layered fiber matrix. The introduction of this multi-scale attribute reproduces the complexity of the hierarchical organization of natural ECM from nanoscale to microscale. In order to better understand the gel dynamics and network characteristics of the obtained hydrogels, the researchers analyzed their gel process and viscoelastic properties (Figure 2b-i~iv). Before visible light irradiation (20min), NorHA, NorHA protein (ie without coagulant) and PL-NorHA (ie with coagulant) have lower storage modulus, and their mechanisms are similar (G≈1-2Pa and G≈0.4-1Pa). However, when exposed to visible light, due to the covalent photo-crosslinking reaction of the thiol-ene reaction, the elastic modulus and viscous modulus increase and reach the storage modulus plateau within a few seconds (2b-i-iv) . PL-NorHA hydrogel (G: 593±156Pa-1wt.% and G: 3536±159Pa-2wt.%) contains only NorHA (G: 1655±238Pa-1wt.% and G: 4845± 376Pa-2wt.%) or NorHA hydrogels containing protein (G: 1561±75Pa-1wt.% and G: 5324±722Pa-2wt.%) have lower storage modulus.
2. Release of protein in PL-NorHA hydrogel. The biosynthesis and tissue turnover of HA are regulated by synthetase and hyaluronidase. The researchers used the quantification of uronic acid or total protein in the hydrogel to draw the in vitro degradation curve of the hydrogel (Figure 2C). Studies have found that in the presence of hyaluronidase, the total degradation time and degradation rate of the hydrogel are faster. There is also a correlation between the degradation time and the crosslink density. The degradation rate of high concentration (2wt.%) is slower than that of low concentration (1wt.%). In addition, the degradation rate of PL-NorHA hydrogel is faster than NorHA (Figure 2c-i,iii). This is because PL-NorHA hydrogels, in addition to the covalent photo-crosslinked network (NorHA), also contain a cross-linked PL protein-bound fiber matrix. Therefore, the degradation of PL-NorHA hydrogel is not only due to the release of uronic acid (representing HA degradation), but also related to the degradation of the fibrin matrix. 1wt.% PL-NorHA hydrogel (92±1%) has higher PL-derived protein release than 2wt.% PL-NorHA hydrogel (72±2%). These results indicate that different combinations of HA macromolecule concentration and fibrin matrix can regulate the release of drugs in drug-loaded hydrogels.
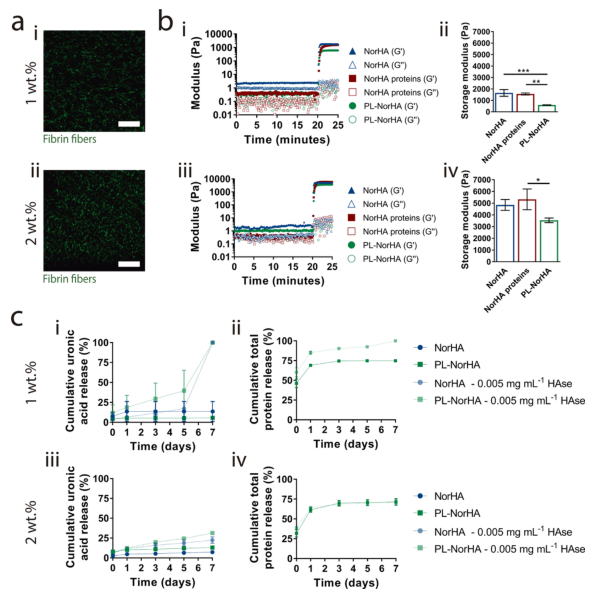
Figure 2 Characterization of the mechanical properties and degradation properties of PL-NorHA hydrogels 3. The in vitro response of MSCs to PL-NorHA hydrogels. Researchers inoculated MSCs cells in NorHA, NorHA-RGD and PL-NorHA under serum-free conditions On the hydrogel, the cell morphology was observed after 4h and 1d in vitro culture. The cells seeded on the NorHA hydrogel usually exhibit a round morphology, with some protrusions at the concentrations of 1wt.% and 2wt.%, showing comparable cell spreading areas and aspect ratios over time (Figure 3a) . The 1wt.% and 2wt.% NorHA-RGD hydrogels can greatly increase the cell spreading area. In PL-NorHA hydrogels (1wt.% and 2wt.%), the cells adhered, spread and presented a spindle-like morphology after only 4 hours of culture (Figure 3a, b). After culturing for 1 day, MSCs showed slender protrusions and formed interconnected networks with neighboring cells. These changes may be caused by the fibrous network formed by PL-NorHA hydrogel. It not only provides a temporary hierarchical extracellular matrix, giving mechanical support and extracellular matrix topology, but also contains many binding domains that mediate interactions with cells, extracellular matrix proteins and growth factors.
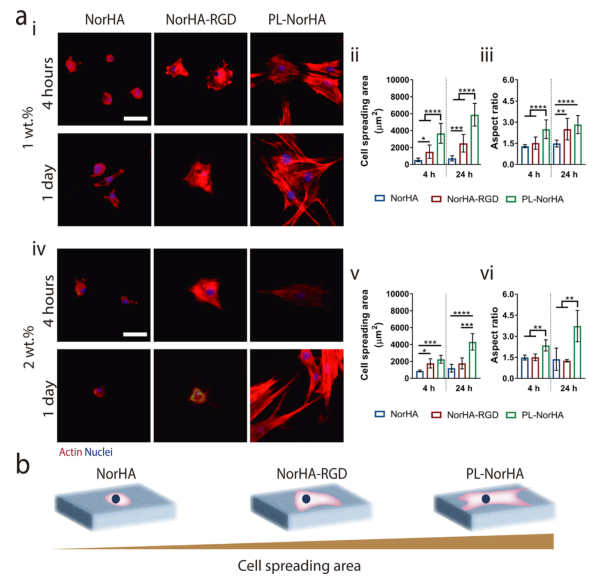
Figure 3 Evaluation of in vitro cell viability of PL-NorHA hydrogel
4. Preparation and characterization of microgels. NorHA (coagulation factor) and PL containing coagulation factors are introduced into the Y-shaped microfluidic chip through two independent channels at different flow ratios, and they are converged into a laminar flow channel. Promote the polymerization of fibrin. Then, this single channel is interrupted by the incompatible continuous phase, producing water-in-oil droplets. When passing through the serpentine path, fibrin further polymerizes and cross-links with visible light at the end of the channel to form a microgel (Figure 4a). Researchers incorporated fluorescein-labeled glucose during the formation of the microgel to analyze its morphology (including size and roundness) (Figure 4b, c). PL-NorHA microgels produced a uniform and layered fibrin network (Figure 4b-ii). The average diameters of the spherical microgels are 120±12μm (1wt.%NorHA), 126±17μm (1wt.%PL-NorHA), 100±8μm (2 wt.%NorHA) and 119±21μm (2 wt.%). PL-NorHA) (Figure 4c-i,ii). Through the release of uronic acid and total protein, the researchers assessed the degradation of the microgel. Studies have found that microgels show a tendency to degrade in the presence of hyaluronidase, and that microgels with low concentrations degrade faster than microgels with high concentrations; PL-NorHA microgels degrade faster than NorHA microgels The gel is faster; the degradation rate of the microgel state is faster than that of the hydrogel state (Figure 4d-i, iii). The total protein release was quantified. It was found that 7 days later, with or without hyaluronidase, the total protein release of PL-NorHA was 41%±7% and 69%±3%, respectively. In summary, it is shown that the stability of the microgel and the release rate of treatment-related proteins can be adjusted by changing the cross-linking density and the concentration of hyaluronidase.
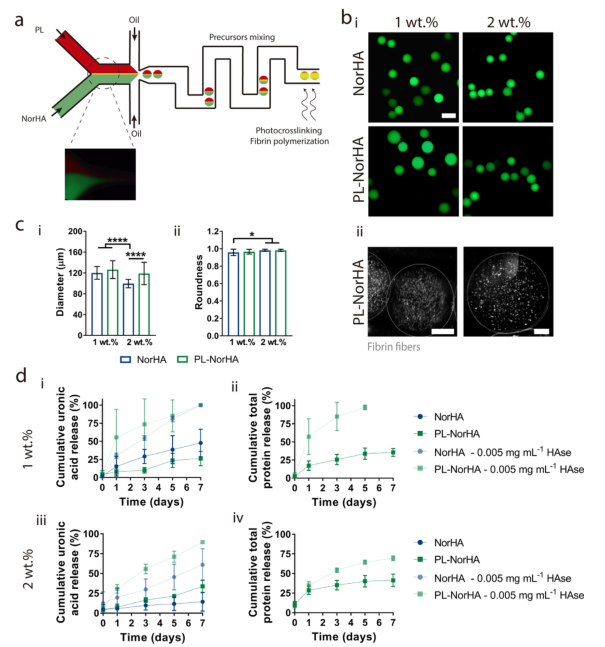
Figure 4 Preparation and characterization of PL-NorHA microgel
5. The rheological properties of the particulate hydrogels In order to study the printability of the microgels, the researchers evaluated the rheological properties and structure of the microgels (Figure 5). The experimental results of oscillating shear rheology show that when the concentration of NorHA polymer in the microgel increases from 1% to 2%, the storage modulus of the particulate hydrogel increases by an order of magnitude (Figure 5a-I, iv). Consistent with the observations in the hydrogel, the presence of PL significantly reduced the storage modulus of the granular hydrogel (from 77±3Pa to 234±2Pa, 2wt.% NorHA and PL-NorHA, respectively). The microgel has shear thinning and self-healing rheological properties, and can be extruded with minimal force without changing the initial properties. The granular hydrogels prepared from different concentrations of microgels (1wt.% and 2wt.%) all showed shear yield with increasing strain (Figure 5a-ii, v). Through a series of high (500%) and low (0.5%) strain scans, the self-healing behavior of all particle hydrogels was observed. After being subjected to high strain, the material showed rapid recovery of solid-like behavior (Figure 5a-iii, vi). In order to better observe the structure of the particulate hydrogel, the researchers incubated the microgel with rhodamine thiol for visualization before making water-in-oil droplets. The results show that 1wt.% NorHA and PL-NorHA microgels show very small porosity, while 2wt.% PL-NorHA microgels increase the porosity, and 2wt.% NorHA microgels have the largest porosity. This may be due to the viscoelasticity of the microgel. The low mechanical extrusion of microgels increases their bulk density and reduces the surface area (Figure 5B).
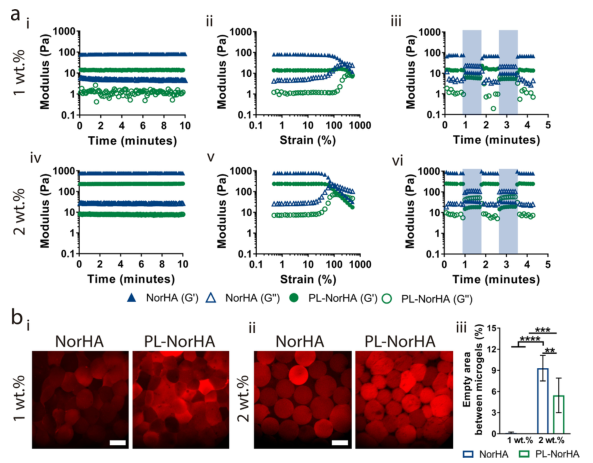
Figure 5 Rheological and morphological characterization of PL-NorHA granular hydrogel
6. 3D printed particle hydrogel and its in vitro cell response In order to evaluate the printability of the microgel ink, the researchers printed the microgel containing FITC-dextran on the surface of the glass slide in filament form, and used fluorescence Microscopic observation (Figure 6a). Due to its granular structure, the microgel ink is easily extruded to form uniform filaments, presenting a densely packed microgel morphology. Furthermore, the researchers used microgel inks for 3D printing to create 3D structures (Figure 6b-i). The lower concentration of microgel ink produces a stable 3D structure (Figure 6b-ii, iii, v), and the higher concentration of NorHA microgel produces a structure that is easily destroyed (Figure 6b-iv). In addition to structural integrity, the printed structure also shows high fidelity. Then the researchers used 1wt.% NorHA and PL-NorHA microgels loaded with MSCs cells for 3D printing. MSCs grown on NorHA microgels showed a round shape (Figure 6c-i), similar to the results obtained from NorHA hydrogels; while the interconnected micropore spaces in PL-NorHA microgels promoted MSCs cells The massive migration and the formation of a 3D cell network (Figure 6c-i, iii). This indicates that PL-derived proteins can promote cell adhesion and survival, and can form a hierarchical fibrin network that can provide cell anchor points.
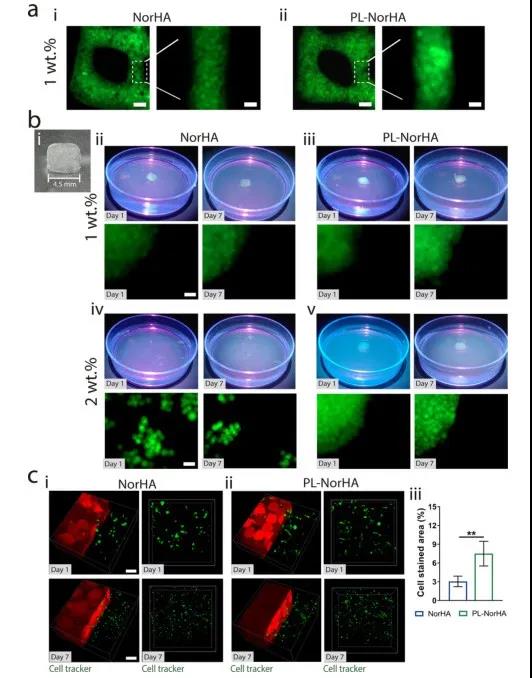
Figure 6 3D printing structure stability and in vitro cell viability evaluation of PL-NorHA microgel.
7. Summary The researchers photo-crosslinked norbornene-modified hyaluronic acid (NorHA) and human platelet lysate (PL) to prepare PL-NorHA hydrogel ink. PL-NorHA hydrogel ink can adjust the release of PL-derived proteins by changing the concentration of HA, and the formed hierarchical fibrin network can promote the adhesion and migration of MSCs cells. In addition, the PL-NorHA microgel ink constructed based on microfluidic technology shows shear thinning and self-healing properties, and can be used for minimally invasive drug delivery and 3D bioprinting.
18915694570
Previous: After Nature, AM will